The use of Allergoids and Adjuvants in Allergen Immunotherapy
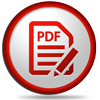
Celso Eduardo Olivier*
Instituto Alergoimuno de Americana, São Paulo, Brazil
*Address for Correspondence: Celso Eduardo Olivier, MD PhD, Instituto Alergoimuno de Americana, Rua Cuba, 836-Bairro Frezzarin-Americana, São Paulo, CEP 13465-773, Brazil, Tel: +55 19 3461-6669, Email: celso@alergoimuno.med.br
Dates: Submitted: 14 August 2017; Approved: 18 September 2017; Published: 20 September 2017
How to cite this article: Olivier CE. The use of Allergoids and Adjuvants in Allergen Immunotherapy. Arch Asthma Allergy Immunol. 2017; 1: 040-060. DOI: 10.29328/journal.aaai.1001006
Copyright License: © 2017 Olivier CE. This is an open access article distributed under the Creative Commons Attribution License, which permits unrestricted use, distribution, and reproduction in any medium, provided the original work is properly cited.
Keywords: Allergens; Allergoid; Allergists; Bioengineering; Hypersensitivity; Immunologic desensitization; Immune system; Polymerization; Precision medicine

Allergen immunotherapy (AIT) is the unique curative treatment to help allergic patients to get over their allergies. With a personalized approach, AIT is the best example of precision medicine. After a century of intensive studies and innovative discoveries, allergists have in their hands many tools to orchestrate the best strategy to re-educate the hypersensitive immune systems that decrease the quality of life of their patients. This review describes both the historical and the promising acquisitions in this field, focusing the biochemical and Bioengineering tools that render an allergen more suitable for a secure, convenient and effective immunotherapy.
Allergen immunotherapy (AIT) is the unique curative approach to treat allergic diseases [1]. Since the seminal work published by Curtis in the New York Medical Record of July 13, 1901 employing “drops” of tincture or fluid extracts of pollens prepared by a botanist, several strategies have been employed to induce tolerance to specific allergens in order to improve the quality of life of allergic patients [2]. The experimental induction of oral tolerance in laboratory rodents followed the clinical assays and began its first steps in 1909 [3]. The further steps were to translate the controlled laboratory experiments to the real diversified human clinical disease management [4]. One century of laboratory and clinical experience have shown us that the choice for a tolerance-induction strategy may rely on a more dose-controlled antigen administration as provided by subcutaneous shots, or may rather explore a more cost-effective tolerogenic route, like the sublingual/oral administration, that may induce tolerance by several mechanisms along with low or high doses [5,6]. Usually, to avoid the undesired side effects of its natural reactivity, the AIT is planned with incremental doses of the same unmodified offending allergens that are extracted and isolated from similar sources that originated the clinical symptoms, the so-called allergen-specific immunotherapy (ASIT) [7]. This is in contrast with the so-called non-specific desensitization that employs correlate antigens or simple microorganism-like unspecific adjuvants to modify the Th1/Th2 balance and immune deviation [8,9]. To produce desensitization, the initial administered dilutions must not elicit allergic reactions and, as the ASIT progresses with increased concentrations of allergens, the induced tolerance mechanisms suppress the hypersensitivity reactions, allowing the prescription of higher concentrations of the natural allergens [10]. Therefore, the main limitation of ASIT is its intrinsic allergenicity derived mainly from its conformational epitopes recognized by specific antibodies, while its therapeutic (immunogenic) activity derives from its hidden linear epitopes, recognized by T regulatory (Treg) cells after proper intracellular antigen-presenting cell (APC) digestion and intercellular class II major histocompatibility complex (MHC) presentation to the T cells receptors (TCR) [11]. The presentation of the linear epitopes to the Treg cell in a non-inflammatory context induces the production of regulatory cytokines such as IL-10 and TGF-ß with further decrease of the B cell production of reaginic antibodies (such as the IgE), stimulation of the regulatory B cells (Breg) and increase of the production of blocking antibodies (such as the secretory IgA and IgG4) [12-16]. Blocking antibodies act competing with reaginic antibodies [17]. Secretory IgA acts on mucosal surfaces ensuring immune exclusion and immune modulation [18]. IgG4 promotes tolerance due to its functional bivalence and incapacity to fix complement [14,19]. However, the administration of allergens in an inflammatory context, as it occurs with the progression of the subcutaneous shots, also stimulates the production of the less pathogenic Th1 cytokines, a fact that may be beneficial for the treatment of Th2 allergic diseases but can theoretically induce an undesirable T cell reactivity [20]. In order to decrease the unpleasant collateral effects of ASIT derived from its intrinsic allergenicity the medical community conceived the production of allergen derivatives, that are generically known as allergoids [21]. An allergoid is a modified allergen designed to have a reduced allergenic activity, when compared with the native allergen, along with a sufficient immunogenic activity [22]. The use of allergoids was inspired in the work of Lowenstein (1909) who inactivated the toxic activity of the tetanus toxin by the use of formaldehyde, producing an immunogenic non-toxic derivative [23]. The first claimed conception for the use of allergoids for allergic desensitization was registered by Carter, from Abbot Laboratories, in the US patent 2,019,808 (1935), where he described the techniques to produce detoxified pollen extracts. Since then, the clinical benefits and safety of the use of allergoids in AIT strategies have been demonstrated in several double-blind placebo controlled clinical trials [24]. The use of allergoids in an AIT tolerance-induction strategy is rationalized by the decrease in number of conformational B cell epitopes recognized by IgE reaginic antibodies (less allergenicity) and maintenance of the IgG epitopes and linear T cell epitopes (immunogenicity) [25-28]. The first studies about allergoids demonstrated that they did not combine with cell-bound IgE (i.e. showed no “hapten-like” activity) due to their incapacity to inhibit the histamine release from leucocytes [29]. In fact, long-term clinical studies performed with specific subcutaneous allergoids demonstrated a low risk of serious adverse reactions [30]. The conception that the allergenic conformational epitopes may not be involved in the mechanisms for desensitization leads to the understanding that, in a tolerance induction strategy, it is not necessary to employ all the immunoreactive epitopes of a given specific allergen [31]. The use of one or few immunodominant T cell linear epitopes is able to produce tolerance to the original allergen and even to related allergens inducing the so-called linked epitope suppression [32]. However, the fact that the allergoid has a diminished IgE-mediated reactivity does not exclude the possibility of a hypersensitivity reaction mediated by a non-IgE mechanism such as the type IV of Gell and Coombs [33]. The great challenge to produce more convenient allergens to the clinical management of allergic diseases stimulated the scientists to develop a molecular approach to a better understanding of the in vitro and in vivo mechanisms of allergenicity and immunogenicity [34]. In order to develop a product for a tolerance-induction strategy it is convenient that the scientist and the practitioners possess objective tools to evaluate the allergenicity and the immunogenicity of the modified antigens, that are candidates to be used in clinical settings [35]. Several clinically accessible tools can evaluate allergenicity such as specific IgE dosage, the allergic skin tests, the provocation tests and the basophil degranulation tests [36,37]. The humoral and cellular immunogenicity of a given allergoid may generically be evaluated by the final production of allergic-specific blocking antibodies or with help of cellular ex vivo tests, such as the dosage of Th1/Th2/Treg cytokines, the lymphocyte proliferation assay, the leukocyte adherence inhibition test, the leukocyte migration inhibition test or the degradome assay [38-43]. Some allergoids have sufficient immunogenicity to be used alone, but some ones, specially the short ones do not have, per se, enough immunogenicity, what raises the need of the association with adjuvants, such as proteic carriers and immune stimulants to amplify the desired immune response [44]. The safety and efficacy of allergoid immunotherapy turned it, now a day, a real tool for desensitization [45]. The lack of allergenicity of allergoids allows their use in accelerated up-dosing schemes, as well ultra-short-course boosters, shortening the immunotherapeutic process [46-49]. We can find in the literature different ways to produce allergoids and adjuvants. In this review, we expose some of these techniques.
Extraction and purification methods
The first AIT preparations were done with help of physical methods such as freezing, thawing, boiling and dilution (in distilled water or salt solutions) of the natural sources related with the patient’s symptoms, in order to “extract” the active constituents whose allergenicity was further corroborated by conjunctival and/or skin tests [50]. One of the very first problems to be solved was the dilution of the extract, since the first undiluted solutions originated severe reactions that prevented the continuity of the treatment [51]. Allergologists also realized that only one or few components of the allergen’s extract were responsible for the symptoms, and with help of purification methods, they began to isolated proteins to perform the diagnosis and the specific immunization [52]. Soon, several extraction solutions, idealized to alter the solution’s pH, and therefore the ionic liaisons between the constituents, began to be employed in the preparation of the extract [53]. Similarly, additional solutions were idealized for further dilution and conservation of the diagnostic and therapeutic preparations, such as the glycerin, a good preservative agent with the inconvenient to produce pain at the injection site at larger concentrations [54]. Besides preservation, high concentrations of glycerin have the capacity to inactive catalytic activity of allergens such as molds and insects, allowing their combination without loss of immunogenicity [55]. Initially, allergists employed only nonstandardized personalized allergen’s extracts but now a day, standardized, commercially available and ready-to-use preparations, with one or multiple allergens were added to the clinical arsenal [56]. When the extraction solution also submits the allergen’s extract to an acid and/or alkali treatment and concomitant dialysis in order to remove substances attached to the proteins by ionic liaisons, the process is called depigmentation [57]. Depigmentation significantly decreases the enzymatic activity and the allergenicity of active extracts [58]. Now a day, depigmentation is considered a preliminary step to further process of the AIT preparations [59].
Allergen modifying processes
Thermal Denaturation: Cooking is an universal, ancient and domestic way to produce allergoids [60]. Thermal processing of proteins can potentially denature some of their conformational epitopes, decreasing their allergenicity and keeping their immunogenicity by the concomitant preservation of the linear epitopes [61]. Additionally, thermal processing may render thermolabile allergenic proteins more susceptible to peptic digestion [62]. Thermal denaturation is used in the desensitization of cow’s milk-allergic patients [63]. However, heat denaturation may also expose new antigens by unfolding the proteins, enhancing their allergenicity [64].
Chemical Denaturation: In the era before the academic knowledge of the T-cell, formalin, tannic acid and other chemicals were used by allergists to denature and precipitate pollen’s extracts in order to reduce their “immediate reactivity” while keeping their “antigenicity” [65]. After the discovery of T-cell’s properties, allergists keep studying others chemical denaturation methods such as urea, as a way to reduce antigen’s allergenicity while keeping its immunogenicity in order to originate safer products to “hyposensitization treatment” [66].
Chemical Polymerization: The formalin’s properties, earlier used to denaturize and precipitate pollen’s extracts, lead to further studies that demonstrated that the formalized extracts have a high molecular weight due to chemical polymerization, originating the conceptualization of the allergoids [29]. In preliminary studies, the most promising agents to produce allergoids by chemical polymerization were the aldehydes, mainly formaldehyde and glutaraldehyde [67]. The aldehydes react with the primary amines, producing covalent cross-links between the proteins while preserving some of the immunological and enzymatic properties of the original extract [68,69]. This concept was largely explored since it was observed that polymerized allergoids were significantly less reactive than the native extracts, while retaining their capacity to induce blocking antibodies in guinea pigs [70]. Glutaraldehyde polymerized extracts of grass pollen and house dust mite significantly increased the ratio of regulatory-to-effector T cells in peripheral blood mononuclear cells (PBMC) cultures of allergic humans to these antigens when compared to the native extracts [71]. Meanwhile, in allergic volunteers, skin tests demonstrated the reduction on allergenicity produced by polymerization [72]. Clinical studies demonstrated the opportunity of chemically polymerized allergoids to be used as a tool for allergic desensitization [73]. The first allergens submitted to chemical polymerization were the pollen’s extracts, followed by mite’s extracts and food allergens such as albumin [74-80]. Recently, glutaraldehyde polymerization is being accomplished also with cat dander [81]. Comparing the polymerization produced by the aldehydes, glutaraldehyde apparently had a stronger effect than formaldehyde, since its polymers are less allergenic, but possess also a lower T-cell stimulatory capacity [82]. The process of polymerization (chemical or enzymatic) may be facilitated by the use of additives, usually amino acids [29,83]. The polymerization process generates relatively great molecules, from the order of 105 to 106 Da [84]. This increase in the molecular weight prevents its absorption by the mucosal membranes, further systemic circulation and occasional allergic reactions [85]. This peculiarity turns the polymerized allergoids particularly attractive for use in sublingual preparations once the first pass to tolerization is the internalization by phagocytosis/pinocytosis of the antigens by the submucosal DCs [86]. To increase the DC intake and subsequently improve the T-cell stimulatory capacity, glutaraldehyde-generated allergoids may be covalently coupled with mannan, a fungi polysaccharide with a pathogen-associated molecular pattern (PAMP) activity able to link to several pattern recognition receptors (PRRs) [87]. Now a day, chemically polymerized allergoids are commercially available to contribute with the tolerance-induction strategy in the treatment of allergic patients [88-91].
Carbohydrate conjugation
There are several simple techniques to couple carbohydrates to allergenic proteins and allergoids. The resulting neoglycoproteins loose several functional activities from the original molecules, such as enzymatic activity and IgE binding capacity. The coupling with preserved innate antigens such as mannan has the additional property of increase the capture of the molecule by the dendritic cells [92].
Enzymatic polymerization
Food industry has employed, for a long-time, cross-linking microbial enzymes as an addictive to increase the consistence of dairy products by means of polymerization [93]. The transglutaminase (TG) produced by the Streptoverticillium mobaraense is commercially available to produce culinary products either at a industrial level in the finalization of whey cream cheeses and yogurts, or at a handcraft level in Japanese restaurants where the chefs use it as an edible glue for foods [94]. One of the main activities of TG is the catalysis of the covalent link between the ε-amino group of lysine with the γ-carboxamide group of glutamine resulting in resistant intra-molecular and inter-molecular proteic bridges and polymerization [95,96]. Transglutaminase is a multifunctional enzyme present in most living organisms, including the human being [97]. Submitted to the bioavailability and immunoreactive tests recommended by the FAO/WHO to approve food addictives, the microbial TG was susceptible to peptic digestion and did not present amino acid sequences coincident with any known food allergen [98]. The modifications induced by TG in the allergenicity and immunogenicity of bovine beta-lactoglobulin are yet at pre-clinical studies but have already demonstrated by in vitro, in vivo and ex vivo analyses promising results for the employ of TG polymerized food proteins as allergoids to treat allergic patients [40,99-101]. The TG repolymerization of hydrolyzed fragments of cow’s milk proteins also produced a great reduction on immunoreactivity [102].
Chemically modified monomeric allergoids
Monomeric allergoids are allergens with punctual modifications at strategic sites that do not produce polymerization and preserves the T-cell linear epitopes [103]. Mite, pollens, cat epithelia and food allergens have already been chemically modified in order to lower their capacity to bind specific IgE [104]. The most clinically employed method uses potassium cyanate (KCNO) to transform the lysine’s ε-amino group into ureido-groups [105]. These carbamylated allergens demonstrated a lower in vitro and in vivo allergenicity while preserving immunogenicity as demonstrated by the capacity to induce IgG antibodies able to react with the native antigens [106]. Carbamylated allergoids have proved safe and effective in the treatment of allergic conditions [107]. This strategy is already commercially available, mainly in Europe as sublingual tablets for treatment of dust mite and/or grass-pollen allergic patients [108-111]. Other methods such as the succinylaton are yet at pre-clinical study [112].
Allergen peptide immunotherapy
The reduction of three-dimensional complex proteins to simpler linear peptides is the first physiological gastrointestinal mechanism to reduce food protein allergenicity. However, alone, it is not always enough to provide a sustained tolerance to the dietary antigens [113]. The intracellular digestion that occurs in dendritic cells (DC) also promotes the destruction of the conformational epitopes and generates free linear epitopes presented to Treg cells to promote immune tolerance. Peptide immunotherapy can provide not only a hypoallergenic provision of T-cell linear epitopes to a tolerance-induction strategy but also a supply of short monovalent B-cell epitopes unable to produce cross-links between antibodies [114]. These peptides, able to bind to specific antibody’s paratopes (competing with the original antigen’s epitopes) are called “mimotopes” [115]. The saturation of the reaginic paratopes with monovalent mimotopes prevents the cross-link between reaginic antibodies fixed to the surface of the effector cells, blocks the signaling to the allergic reaction and produces a state of temporary competitive desensitization. That is also the mechanism explored during mast cell desensitization to treat drug hypersensitivity [116]. Aspirin desensitization is an example of mimotope desensitization. As a small molecule, with an average mass of 180Da, aspirin by itself is not able to cross-link antibodies, but when conjugated to circulating proteins it becomes an allergen. Aspirin desensitization is done by slow saturation of antibodies fixed at effectors cells surface, allowing a temporary state of tolerance [117]. Eventually the tolerogenic administration of a hapten may also induce a permanent state of tolerance if the resulting hapten-conjugate protein is able to induce cellular and/humoral mechanisms to block the hypersensitivity, as it is achieved in nickel desensitization [118,119]. Therefore, peptide immunotherapy may provide two distinct mechanisms to treat allergic patients. The first mechanism is a tolerogenic Treg-orchestrated desensitization (tolerogenic desensitization) that generates tolerogenic cytokines (IL-10, TGF-β) as well B cell-induced blocking antibodies (IgA and IgG4). The second mechanism is a competitive mimotope desensitization (competitive desensitization) that competitively blocks the encounter of the original allergens with the reaginic antibodies preventing cross-linking and the subsequent allergic reaction [120]. Once proved that the allergenicity of the mimotopes are clinically insignificant in a given patient, the concentration of the initial peptide immunotherapy may be relatively greater than the concentration of natural allergens as would be employed in an ASIT strategy. So, in a combinative AIT with allergoids and natural antigens, the participation of allergoids can be initiated with higher concentrations, followed by a gradual increase of the unmodified allergen concentrations. This strategy will explore the competitive desensitization promoted by the mimotopes along with an increasing stimulation of the Treg tolerogenic mechanisms. Besides allergen immunotherapy, mimotopes are tools also used for a great variety of applications such as epitope mapping and cancer immunotherapy [121]. Peptide immunotherapy may be accomplished in two ways: enzymatic hydrolysis and biological engineering.
Enzymatic hydrolysis
Peptic digestion is the first physiologic barrier to prevent food allergic diseases [122]. Pepsin is a gastric protease that naturally renders tolerable most allergenic proteins that otherwise would produce allergic symptoms [123]. So do trypsin and others proteases and peptidases found in small intestine [124]. The in vitro use of a simulated gastric juice is an established method to study and predict protein’s allergenicity, since the more resistant to peptic digestion, the greater the possibility to produce sensitization and allergy [125]. The oral allergy syndromes, induced by pepsin-sensitive allergens such as pollens and fruits demonstrate this concept, since they usually produce local symptoms before the gastric passage, but may occasionally produce systemic symptoms when the digestive function is compromised by some disease or by the use of antacids [126-128]. The hydrolysis produced by mean of the use of different proteases is a strategy to reduce allergenicity of clinically relevant proteins. A given protein may originate different hydrolysates according with the protease employed [129]. Additionally, the oral use of partially hydrolyzed (but not extensively hydrolyzed) proteins was able to induce tolerance to egg’s and cow’s milk proteins in animal models [130-133]. The tolerogenic effect is dependent of the presence of the T-cell epitopes inside the peptide sequence of the hydrolysate and related to the reduction of both serum histamine and IgE, the increased intestinal expression of TGF-β and FOXp3, as well the inhibition of both Th1-biased (IFN-γ and IL-12) and Th2-biased (IL-4 and IL-13) cytokines [134]. However, differently of the native proteins, a casein extensive hydrolysate alone was not able to stimulate a humoral or cellular response in human babies [135]. The association of allergoids produced by peptic digestion with unmodified allergens can also be used as a strategy to induce tolerance to pepsin-sensitive allergens [8].
Biological engineering
When designing an antibody-guided immunotherapy, the departure point is the choice of the best protective epitope. Diversely from the immunotherapies extracted from the natural sources presenting a great variety of immunogenic epitopes, the engineered immunotherapy will usually deal with just one or a few molecules to produce an epitope-based vaccine [136]. So, the choice of the immunotherapeutic peptides is crucial, mainly due to the high costs of the methodologies. According to the technique employed, Bioengineering produces two kinds of peptides: the synthetic and the recombinant. Several software tools based on matrix algorithms assembled from X-ray crystallography data were developed to aid the design of immunotherapeutic peptides [137]. This “in silico” approach helps to improve the prediction of class I and class II MHC epitopes before the benchwork [138]. Synthetic peptides have been investigated as vaccine candidates for over 40 years [139]. Bacteriophage-based techniques, such as phage display peptide libraries are also valuable tools for epitope mapping, identification, validation and production of mimotopes in suitable amounts for allergen immunotherapy [121].
Synthetic peptides
The design of a T-cell-specific reactive peptide begins with the mapping of T-cells from allergic patients. The first technique for the sequential production of a designed synthetic peptide was developed by the Nobel Chemistry laureate Robert Bruce Merrifield in 1963 [140]. The first method to assemble a synthetic peptide with the resolution of a single amino acid in order to be used as an epitope was developed in 1984 and gave birth to the epitope mapping and assemblage techniques in solid phase such as the Pepscan, as well purification techniques to assure a proper immunogenic molecule [141-143]. Regarding to their use in the immunotherapy strategies, allergen-derived peptides are classified in long, short and overlapping peptides. The greater the peptide higher is its immunogenicity and allergenicity. Unfortunately, small peptides may not elicit strong immune responses, what brings the need of immunogenic carriers and adjuvants for some of them [139]. Long synthetic peptides (over a hundred residues) were designed to reproduce the entire sequence of the allergenic protein and cover all possible T-cell epitopes. The use in clinical trials of long synthetic peptides in the treatment of allergic patients demonstrated to be safe (despite some mild immediate and late reactions), produced Th1-type immune deviation, allergen-specific IL-10 production and T-cell hyporesponsiveness [144]. The residual side effects of the long synthetic peptides stimulated the employ of short synthetic peptides. Successful proof-of-concepts were described in the treatment of cat-allergic and bee venom-allergic patients with short synthetic peptides that were able to down regulate the allergic response to the whole protein [145-147]. However, the administration of Fel d 1 short synthetic peptides (even with only 27 residues of amino acids) was able to produce immediate and late allergic symptoms in a small number of patients. It was demonstrated by ex vivo experiments, that under high dosage stimulation, allergen-derived short peptides act directly (in the co-stimulatory presence, but not by intermediation of APC) on T cells with multiple TCR. This direct action on TCR decreases the production of TH2 cytokines such as IL-4 but may be responsible for the Th1-biased late reactions [148]. This lead to the conception of the overlapping T-cell epitopes peptides: shorter peptides (16-17 residues) sectioned from the main epitope with overlapping sections encompassing the entire epitopes sequence. The administration of a mixture of 12 Fel d 1 overlapping peptides did not produce immediate allergic reactions, but was able to produce late asthmatic reactions in a dose-dependent fashion and further tolerance to subsequent injections. These late reactions were associated with cutaneous late-phase reactions to whole cat dander [149]. Contiguous overlapping peptides are also being investigated for birch pollen AIT [150-152].
Recombinant peptides - isoallergens
Genetic engineering, by means of diverse laboratory techniques is able to produce homologous and heterologous pieces of recombinant DNA (rDNA) with punctual alterations that produce proteins and/or peptides with modified properties from the natural source [153]. The detailed characterization of the DNA/RNA segment responsible for the transcription/translation of a specific allergen allows to the investigators to produce, with help of the Escherichia coli transcription apparatus, grams amounts of original and modified peptides in order to select hypoallergenic derivatives (isoallergens) to employ for modulation of allergic reactions [154]. The use of these modified peptides in asthma mice models produced a decrease in the inflammatory infiltrate that suggests a competitive desensitization, independent of an additional Treg tolerance induction with blocking IgG production provided by the long-term use [155]. Recombinant allergens may present several occurring isoforms. The IgE-binding capacity of these isoforms may differ, what result that some isoforms may be more allergenic, while some isoforms may be more immunogenic. One of the most studied isoallergens is the recombinant Bet v 1, the major allergen of birch pollen. The allergenicity and immunogenicity of natural and recombinant isoforms of Bet v 1 was evaluated by immunoblot, skin tests and T cell activation. The recombinant d isoform of Bet v 1 showed to be less allergenic and more immunogenic than the natural and the recombinant a isoform of Bet v 1 [156]. The higher immunogenic capacity of the d isoform of recombinant Bet v 1 was attributed to its natural trend to aggregation [157].
Carrier bound peptides
The use of small peptides for AIT is convenient to induce mimotope competitive desensitization, but their immune hyporesponsiveness may not induce proper Treg orchestrated tolerogenic desensitization. To solve this problem some investigators coupled epitope-based small peptides with greater molecules that acted as a carrier to stimulate the innate processing of the peptide and a proper presentation to naive T cells in a tolerogenic environment. One of these carriers was the keyhole limpet hemocyanin, a metalloprotein that bounded to Der p 2 peptides induced specific IgG antibodies that inhibited allergen-induced basophil activation in a similar way that the native allergen, without the inconvenience of the side-effect produced by IgE-mediated allergic reactions [158]. In a similar way the B subunit of the cholera toxin conjugated with ovalbumin, administered prior to the sensitization, lead to a decrease of antigen-specific IgE and an increase of antigen-specific IgG2a when compared to non-pretreated sensitized animals [159]. Another interesting fusion was the couple of grass pollen’s epitope-based peptides with the PreS proteins from Hepatitis B virus (HBV). This conjugate was produced in large amounts by recombinant expression in Escherichia coli and when applied to animals and humans was able to induce not only allergen-specific IgG antibodies, that inhibited the patient’s IgE binding to the grass pollen allergens, but also HBV-neutralizing antibodies [160].
Hybridization
Also, through genetic engineering, by means of Polymerase Chain Reaction (PCR) researchers are able to combine heterologous T cell epitopes from similar allergens into a unique hybrid molecule suitable for use in AIT. The engineered hybrid allergoid may be projected to have a peculiar conformational structure facilitating the uptake and processing by the dendritic cell, along with an insignificant allergenicity allowing its use in tolerance-induction strategies to treat polysensitized patients [161].
Adjuvants
Adjuvants are substances aggregated to an AIT strategy that modify the way the antigens are processed by the immune system [162]. Adjuvants may act as immune stimulants increasing the innate processing of the antigen (dendritic cells target adjuvants), or may have just a depot-effect (adsorptive adjuvants), simply increasing the permanence of the immunotherapeutic agent on the site of the injection allowing more time for the innate immune system to process the antigens. Sometimes these effects are associated and inseparable. The primary function of an adsorptive adjuvant is not just influence immunocompetent cells, but it is rather intended to exert a short-term or long-term depot effect, reducing the bioavailability of the subcutaneous allergenic extract. This effect is produced by precipitation of the antigen extract that slows its release and allows the use of fewer shots, optimizing the immunotherapeutic effect of each injection. Some adjuvants are employed by their intrinsic immunogenicity, such as the toll-like receptors (TLR) agonists that stimulate the tolerogenic dendritic cells and increase the internalization of the immunotherapeutic extract and further interaction with T-reg cells [163-165]. Among them, the C-type lectins ligands are the most attractive due to their role in allergic sensitization [166]. Lectins are a ubiquitous class of protein domains that share the property to bind to carbohydrates. The denomination C-type lectin refers to the lectins that need calcium to bind to the carbohydrate. Lectins may be found free on the interstitial milieu, where they act as opsonins, or anchored into cytoplasmic membranes where they work as innate receptors [167]. Dendritic cells may be therapeutically targeted with C-type lectins ligands to improve the internalization of the antigens of the AIT preparation [167]. Different adjuvants associated with the same antigen may induce different innate and adaptive immune responses, as well different reactogenicity patterns [168]. Adjuvants may be associated in formulations, especially between adsorptive and dendritic cells target adjuvants, what is now known as Adjuvant Systems [169].
Freund’s Adjuvant: Freund’s adjuvant was originally conceived as a water-in-oil emulsion where the inactivated microorganisms were dispersed to stimulate the innate processing of their antigens. It may be optionally potentialized with mannide monooleate, an emulsifying agent, or with heat-killed mycobacteria, when it is called the Freund’s complete adjuvant [170]. It is not used in human vaccines due to local inflammatory side effects.
Bacterial Lysates: Bacterial lysates inactivated by mechanical, thermal and/or chemical treatment lose their toxicity, but preserve their immunogenicity, acting as immunostimulants. They induce synergistic TLR innate immunity, a shift to production of Th1 cytokines such as type I interferons and Treg and Breg anti-inflammatory cytokines, such as IL-10, that attenuate Th2 allergic responses [171-174].
Aluminum: Salts of aluminum have been employed as immune stimulants since 1924, when it was demonstrated that they potentialized the production of horse’s antibodies against the tetanus and diphtheria toxoids [175]. Their use in the treatment of allergic patients was first described in 1938 [176]. The association of aluminum salts as an AIT adjuvant increases the uptake of the allergens by the antigen-presenting cells and increases the antigen-induced T cell proliferation [177]. To prepare aluminum salts precipitates (alum) for human use it is required a rigorous quality control because minor alterations on particle sizes can modify their properties. Alum was extensively used as a long-term depot-type adjuvant for several decades, however, despite some well-tolerated alum-depot allergen immunotherapies are yet in use; its employ is being gradually abandoned due to reports of toxicity as well immediate and delayed sensitivity [178-184].
L-Tyrosine: L-tyrosine is a non-essential amino acid synthesized in the human body from phenylalanine. L-tyrosine binds non-covalently to the allergenic extract burying its hydrophilic side into the protein’s core, resulting in a hydrophobic derivative [185]. L-tyrosine acts in AIT as a short-term depot-forming adjuvant, decreasing the bioavailability of the formulation, resulting in an indirect increase of the IgG antibody production [186]. The addition of microcrystalline tyrosine to allergoids associated with TLR agonists provides a depot-effect to the AIT formulation [187]. Several different brands of allergoids adsorbed onto L-tyrosine are already in use in Europe for at least 3 decades [188,189].
Mannan: Mannan is a ubiquitous mannose polysaccharide found in plants and fungi that acts as a C-type lectin ligand. The innate immune system has pattern-recognition receptors for mannose-terminated sugars that once activated enhance antigen presentation, DC maturation and immune responses [190]. The addiction of nonoxidized mannan to the glutaraldehyde polymerization of pollen allergens originated a stable allergen-mannan conjugate with low IgE-binding capacity further demonstrated to be a high immunogenic molecule, increasing the production of tolerogenic cytokines, being captured more efficiently by human DCs than the non-conjugated allergoids and the native allergens [191].
Monophosphoril Lipid A: Monophosphoryl lipid A (MPLA) is a hydrolyzed lipid A fraction derivative from lipopolysaccharides (LPS) of the outer membrane of Gram-negative bacteria that works as a TLR-4 agonist [192,193]. The main characteristic of MPLA is its strong immunostimulatory properties and absence of endotoxic effects [194]. This safety/efficacy profile allowed the use of MPLA as an adjuvant incorporated into several commercial vaccines, including AITs with polymerized allergoids [195-200].
Virus-like particles: The innate immune system evolved to recognize viral particles by PRRs such as the TLR-7, that when activated by single stranded viral RNA stimulates the production of Th1 cytokines such as the type 1 interferons, inhibiting the Th2 profile. This property may be advantageously explored in the treatment of type-1 allergies employing virus-like particles in association, or even in the absence of specific allergens (non-specific desensitization). Preclinical trials with a TLR-7 agonist administered by nasal route demonstrated a reduction in the nasal score symptoms after nasal allergen challenge. The main collateral effects were systemic influenza-like symptoms like fever and myalgia [201].
DNA immunostimulatory sequences (CpG): Unmethylated Cytosine-phosphodiester-Guanine (CpG) motifs are TLR-9 ligands that conjugated with bacterial and virus antigens activated Th1 cells, cytotoxic T cells (CTL) and induced the production of gamma-interferon (IFN-γ) and IgG neutralizing antibodies [202]. The conjugation of the ragweed-pollen antigen with DNA immunostimulatory sequences rich in unmethylated CpG motifs, resulted in an immunotherapeutic compound with low allergenicity and increased immunogenicity [203]. These oligodeoxyribonucleotides conjugated with Amb a 1 increased the allergic human PBMC production of Th1 cytokines and decreased the Th2 expression, demonstrating a great immunotherapeutic potential for allergic diseases [204]. Pilot studies employing as AIT strategy injections of conjugates of house dust mite (HDM) or Amb a 1 with immunostimulatory oligodeoxyribonucleotides in allergic patients inhibited the cutaneous and conjunctival responses to sensitizing extracts and alleviated allergic symptoms with good tolerance [205,206].
Lysine Analogs: Lysine analogues act as competitive inhibitors of protein interactions that depend on the lysine residues [207]. Actually, they are indicated for treatment of bleeding disorders due to its competitive suppressing of plasminogen activation by blood and tissue kinases [208]. The ε-aminocaproic acid (an analogue of lysine without the α-amino group) was already used empirically as an adjuvant of SLIT [209]. Theoretically, it has the potential to impair the liaison of the IgE with the lysine-dependent binding sites of the immunotherapeutic extract, lowering its allergenicity. It was extensively used in Brazil for more than 30 years, since the 70’s with the brand name of Aminovac®, commercialized by drugstores under medical prescription. In the early 2010’s it was withdrawn off the market by the sanitary authorities with the explanation that the producer did not fulfill the novel requisites to renew its license.
Vitamin D3
When comparing the capacity of polymerized versus native allergen extracts of grass pollen and HDM to stimulate the proliferation and cytokine production in PBMC of allergic volunteers, the addiction of 1α,25-dihydroxyvitamin D3 reduced effector T cell activation and cytokine production [71]. Further studies demonstrated that Vitamin D3 potentiated the effect of a chemically-modified monomeric allergoid in sensitized mice [210].
Epigenetic Inducers of Foxp3
The expression of FoxP3 in Treg cells depends on DNA demethylation at a conserved CpG-rich region in the FoxP3 locus [211]. DNA methylation is mediated by DNA methyltransferases (DNMTs) which is inhibited by the micro-RNAs encountered in the fat of human breast milk and unprocessed cow’s milk [212]. The milk-derived immune-regulatory exosomal micro-RNAs, particularly rich in the first months of lactation produce demethylation of the FoxP3 locus activating Treg cells [213]. Similarly the intake of raw cow’s milk is also associated with high FoxP3 demethylation and differentiation of Treg cells [214]. The use of FoxP3 inducers is yet just an speculative matter in the field of AIT adjuvants, but justify the incentive to the use of breast milk as prophylaxis for allergic diseases [215].
The science behind the creation of strategies to induce protective immune responses in patients with allergen-induced hypersensitivity reactions had evolved a lot. More than a century of studies revealed many promising approaches that had beneficed an uncountable number of allergic patients. However, most studies and strategies are directed through Gell & Coombs type I hypersensitivity mechanisms which diagnosis is easier and simpler than the other three hypersensitivity reactions. However, the understanding that cellular hypersensitivity precedes the Th2 escalation and that the AIT therapeutic effect does not act directly over IgE, but rather over cellular mechanisms of tolerance, lead us to the believe that the other hypersensitivity mechanisms may also be modulated through AIT [216,217]. Therefore, there is yet a long way to a better understanding of the tolerance mechanisms and the discovery of the ultimate therapy. Meanwhile, the allergic population grows worldwide, requesting a personalized, simple, affordable and quick cure for these debilitating conditions.
- Akdis CA, Akdis Mb. Mechanisms of allergen-specific immunotherapy. J Allergy Clin Immunol. 2011; 127: 18-27. Ref.: https://goo.gl/tfh5HN
- Curtis H. The immunization treatment of hay fever. JAMA. 1902; 39: 1267-1268. Ref.: https://goo.gl/djxtrJ
- Besredka A. De l'anaphylaxie. Sixiéme memoire de l'anaphylaxie lactique. Ann Inst Pasteur. 1909; 23: 166-174.
- Cobbold SP, Li XC. Translating Tolerogenic Therapies to the Clinic. Where Do We Stand and What are the Barriers? Front Immunol. 2012; 3: 317. Ref.: https://goo.gl/5sV5St
- Faria AM, Weiner HL. Oral tolerance. Immunol Rev. 2005; 206: 232-259. Ref.: https://goo.gl/R7ZrtR
- Verheggen BG, Westerhout KY, Schreder CH, Augustin M. Health economic comparison of SLIT allergen and SCIT allergoid immunotherapy in patients with seasonal grass-allergic rhinoconjunctivitis in Germany. Clin Transl Allergy. 2015; 5: 1. Ref.: https://goo.gl/ySkzNy
- Winther L, Arnved J, Malling HJ, Nolte H, Mosbech H. Side-effects of allergen-specific immunotherapy. A prospective multi-centre study. Clin Exp Allergy. 2006; 36: 254-260. Ref.: https://goo.gl/7P6pG1
- Olivier CE, Lima RPS, Argentão DGP, Silva MDd, Santos RAPG, et al. Group-specific Multi-allergen Sublingual/Swallow Immunotherapy Improves the Quality of Life of Polysensitized Children and Adults with Allergic Rhinitis. Journal of Allergy & Therapy. 2013; 4: 148. Ref.: https://goo.gl/3YGw5T
- Klimek L, Bachmann MF, Senti G, Kündig TM. Immunotherapy of type-1 allergies with virus-like particles and CpG-motifsExpert Rev Clin Immunol. 2014; 10: 1059-1067. Ref.: https://goo.gl/8BU7dK
- Cox L, Nelson H, Lockey R, Calabria C, Chacko T, et al. Allergen immunotherapy: A practice parameter third update. J Allergy Clin Immunol. 2011; 127: 1-55. Ref.: https://goo.gl/DMZKZX
- Palomares O, Martin-Fontecha M, Lauener R, Traidl-Hoffmann C, Cavkaytar O, et al. Regulatory T cells and immune regulation of allergic diseases: roles of IL-10 and TGF-beta. Genes Immun. 2014; 15: 511-520. Ref.: https://goo.gl/rRUDoJ
- Bohle B, Kinaciyan T, Gerstmayr M, Radakovics A, Jahn-Schmid B, et al. Sublingual immunotherapy induces IL-10-producing T regulatory cells, allergen-specific T-cell tolerance, and immune deviation. J Allergy Clin Immunol. 2007; 120: 707-713. Ref.: https://goo.gl/9d5zKm
- van Neerven RJ, Knol EF, Ejrnaes A, Würtzen PA. IgE-Mediated Allergen Presentation and Blocking Antibodies: Regulation of T-Cell Activation in Allergy. Int Arch Allergy Immunol. 2006; 141: 119-129. Ref.: https://goo.gl/WEn3nJ
- van de Veen W, Akdis M. Role of IgG4 in IgE-mediated allergic responses. J Allergy Clin Immunol. 2016; 138: 1434-1435. Ref.: https://goo.gl/xBamCk
- van de Veen W, Stanic B, Wirz OF, Jansen K, Globinska A, et al. Role of regulatory B cells in immune tolerance to allergens and beyond. J Allergy Clin Immunol. 2016; 138: 654-665. Ref.: https://goo.gl/RkAGsz
- Noval Rivas M, Chatila TA. Regulatory T cells in allergic diseases. J Allergy Clin Immunol. 2016; 138: 639-652. Ref.: https://goo.gl/KTJB9v
- Till SJ, Francis JN, Nouri-Aria K, Durham SR. Mechanisms of immunotherapy. J Allergy Clin Immunol. 2004; 113: 1025-1034. Ref.: https://goo.gl/aG2qBv
- Favre L, Spertini F, Corthesy B. Secretory IgA Possesses Intrinsic Modulatory Properties Stimulating Mucosal and Systemic Immune Responses. J Immunol. 2005; 175: 2793-2800. Ref.: https://goo.gl/Tvr6jn
- Aalberse RC, Schuurman J. IgG4 breaking the rules. Immunology. 2002; 105: 9-19. Ref.: https://goo.gl/XCMzQS
- Focke M, Swoboda I, Marth K, Valenta R. Developments in allergen-specific immunotherapy: from allergen extracts to allergy vaccines bypassing allergen-specific immunoglobulin E and T cell reactivity. Clin Exp Allergy. 2010; 40: 385-397. Ref.: https://goo.gl/pxwjA8
- Himly M, Carnes J, Fernandez-Caldas E, Briza P, Ferreira F. Characterization of allergoids. Arb Paul Ehrlich Inst Bundesinstitut Impfstoffe Biomed Arzneim Langen Hess. 2009; 96: 61-69. Ref.: https://goo.gl/Kmp9mY
- Maasch H, Marsh D. Standardized extracts modified allergens-allergoids. Clin Rev Allergy. 1987; 5: 89-106. Ref.: https://goo.gl/2DJnDL
- Loewenstein E. Über aktive Schutzimpfung bei Tetanus durch Toxoide. Zeitschrift für Hygiene und Infektionskrankheiten. 1909; 62: 491-508. Ref.: https://goo.gl/XQMVkW
- Bousquet J, Maasch HJ, Hejjaoui A, Skassa-Brociek W, Wahl Rd, et al. Double-blind, placebo-controlled immunotherapy with mixed grass-pollen allergoids: III. Efficacy and safety of unfractionated and high-molecular-weight preparations in rhinoconjunctivitis and asthma. J Allergy Clin Immunol. 1989; 84: 546-556. Ref.: https://goo.gl/ZZ83hP
- Valenta R, Ferreira F, Focke-Tejkl M, Linhart B, Niederberger V, et al. From Allergen Genes to Allergy Vaccines. Annu Rev Immunol. 2010; 28: 211-241. Ref.: https://goo.gl/is9XQy
- Heath M, Bell A, Skinner M, Bullimore AD. Molecular Fingerprinting of Complex Allergoids. Journal of Allergy and Clinical Immunology. 2016; 137: 405. Ref.: https://goo.gl/3hn6tp
- Starchenka S, Bell AJ, Mwange J, Skinner MA, Heath MD. Molecular fingerprinting of complex grass allergoids: size assessments reveal new insights in epitope repertoires and functional capacities. World Allergy Organ J. 2017; 10: 17. Ref.: https://goo.gl/z1eanS
- Heath M, Starchenka S, Bell A, Skinner M. Structural and immunological characterisation of a broad-spectrum grass allergoid vaccine. Journal of Allergy and Clinical Immunology. 2017; 139: 259. Ref.: https://goo.gl/9cLRVL
- Marsh DG, Lichtenstein LM, Campbell DH. Studies on `allergoids' prepared from naturally occurring allergens: I. Assay of allergenicity and antigenicity of formalinized rye Group I component. Immunology. 1970; 18: 705-722. Ref.: https://goo.gl/Ni95rP
- Distler A, Pappelendam D. 13-year overview of serious adverse drug reactions following subcutaneous specific immunotherapy with a chemically modified allergen preparation. Allergo J Int. 2015; 24: 294-302. Ref.: https://goo.gl/XMYanV
- Pickl WF. The Value of Identifying Major T Cell Epitopes of Clinically Important Allergens. Int Arch Allergy Immunol. 2012; 160: 4-6. Ref.: https://goo.gl/UU2kBk
- Campbell JD, Buckland KF, McMillan SJ, Kearley J, Oldfield WLG, et al. Peptide immunotherapy in allergic asthma generates IL-10“dependent immunological tolerance associated with linked epitope suppression. J Exp Med. 2009; 206: 1535-1547. Ref.: https://goo.gl/ksBJbT
- Campana R, Moritz K, Marth K, Neubauer A, Huber H, et al. Frequent occurrence of T cell-mediated late reactions revealed by atopy patch testing with hypoallergenic rBet v 1 fragments. J Allergy Clin Immunol. 2016; 137: 601-609. Ref.: https://goo.gl/SHUYhp
- Ferreira F, Wolf M, Wallner M. Molecular Approach to Allergy Diagnosis and Therapy. Yonsei Med J. 2014; 55: 839-852. Ref.: https://goo.gl/oT5eqW
- Flower DR. Advances in Predicting and Manipulating the Immunogenicity of Biotherapeutics and Vaccines. BioDrugs. 2009; 23: 231-240. Ref.: https://goo.gl/hkWfmH
- Olivier CE, Argentão DGP, Santos RAPG, Silva MD, Lima RPS, et al. Skin scrape test: an inexpensive and painless skin test for recognition of immediate hypersensitivity in children and adults. The Open Allergy Journal. 2013; 6: 9-17. Ref.: https://goo.gl/XHJx2z
- Olivier CE, Argentão DG, Santos Lima RP, Santos RAGP, Fabbri N. Assessment of allergen-induced respiratory hyperresponsiveness before the prescription of a specific immunotherapy. Allergy Rhinol (Providence). 2015; 6: 89-93. Ref.: https://goo.gl/tJcbVM
- Gioacchino MD, Cavallucci E, Ballone E, Cervone M, Rocco PD, et al. Dose-Dependent Clinical and Immunological Efficacy of Sublingual Immunotherapy with Mite Monomeric Allergoid. Int J Immunopathol Pharmacol. 2012; 25: 671-679. Ref.: https://goo.gl/xrDSio
- Iraola V, Gallego MT, Lopez-Matas MA, Morales M, Bel I, et al. Immunogenicity of Phleum pratense depigmented allergoid vaccines: experimental study in rabbits. J Investig Allergol Clin Immunol. 2012; 22: 35-40. Ref.: https://goo.gl/crE4xR
- Olivier CE, Lima RPS, Pinto DG, Santos RAPG, Silva GKM, et al. In search of a tolerance-induction strategy for cow's milk allergies: significant reduction of beta-lactoglobulin allergenicity via transglutaminase/cysteine polymerization. Clinics (Sao Paulo). 2012; 67: 1171-1179. Ref.: https://goo.gl/AS4Ntz
- Egger M, Jürets A, Wallner M, Briza P, Ruzek S, et al. Assessing Protein Immunogenicity with a Dendritic Cell Line-Derived Endolysosomal Degradome. PLoS One. 2011; 6: 17278. Ref.: https://goo.gl/5Ytj7Y
- Thomson DMP. Assessment of immune status by the leukocyte adherence inhibition test. New York: Academic Press; 1982. Ref.: https://goo.gl/CoMKWN
- Vanto T, Smogorzewska EM, Viander M, Kalimo K, Koivikko A. Leukocyte migration inhibition test in children with cow milk allergy. Allergy 1987; 42: 612-618. Ref.: https://goo.gl/gHfAHJ
- Moingeon P. Adjuvants for allergy vaccines. Hum Vaccin Immunother. 2012; 8: 1492-1498. Ref.: https://goo.gl/UbMQjZ
- Häfner D, Gödicke V, Narkus A. Allergen specific immunotherapy has no influence on standard chemistry and hematology laboratory parameters in clinical studies. Clin Transl Allergy. 2014; 4: 18. Ref.: https://goo.gl/qsWcvj
- Buczyłko K, van der Werf JF, Boot D, van Ree R. Accelerated Up-Dosing of Subcutaneous Immunotherapy with a Registered Allergoid Birch Pollen Preparation. Int Arch Allergy Immunol. 2017; 172: 183-186. Ref.: https://goo.gl/ACPT69
- Pfaar O, Lang S, Pieper-Fürst U, Astvatsatourov A, Gerich F, et al. Ultra-short-course booster is effective in recurrent grass pollen-induced allergic rhinoconjunctivitis. Allergy. 2017; 4: 13240. Ref.: https://goo.gl/tHDXJu
- Chaker AM, Al-Kadah B, Luther U, Neumann U, Wagenmann M. An accelerated dose escalation with a grass pollen allergoid is safe and well-tolerated: a randomized open label phase II trial. Clin Transl Allergy. 2016; 6: 4. Ref.: https://goo.gl/Be7XLh
- Morais-Almeida M, Arêde C, Sampaio G, Borrego LM. Ultrarush schedule of subcutaneous immunotherapy with modified allergen extracts is safe in paediatric age. Asia Pac Allergy. 2016; 6: 35-42. Ref.: https://goo.gl/PeD17K
- Noon L. Prophylatic inoculation against hay fever. The Lancet 1911; 177:1572-1573. Ref.: https://goo.gl/v9EHqm
- Cooke RA, Veer AV. Human Sensitization. The Journal of Immunology. 1916; 1: 201-305. Ref.: https://goo.gl/XBU9FK
- Schloss OM. A Case of Allergy to Common Foods. Am J Dis Child 1983; 41:249-252. Ref.: https://goo.gl/VHAoEQ
- Coca AF. Studies in Specific Hypersensitiveness V. The Preparation of Fluid Extracts and Solutions for Use in the Diagnosis and Treatment of the Allergies with Notes on the Collection of Pollens. The Journal of Immunology. 1922; 7: 163-178. Ref.: https://goo.gl/VmhWy6
- Van Metre Jr TE, Rosenberg GL, Vaswani SK, Ziegler SR, Adkinson NF. Pain and dermal reaction caused by injected glycerin in immunotherapy solutions. J Allergy Clin Immunol. 1996; 97: 1033-1039. Ref.: https://goo.gl/fvcFoV
- Daigle BJ, Rekkerth DJ. Practical recommendations for mixing allergy immunotherapy extracts. Allergy Rhinol (Providence). 2015; 6: 1-7. Ref.: https://goo.gl/GQ266R
- Slater JE, Menzies SL, Bridgewater J, Mosquera A, Zinderman CE, et al. The US Food and Drug Administration review of the safety and effectiveness of nonstandardized allergen extracts. J Allergy Clin Immunol. 2012; 129: 1014-1019. Ref.: https://goo.gl/jT6dv2
- Fernández-Caldas E, Gallego M, Carnés J, Iraola V. Enzymatic Activity of Dermatophagoides pteronyssinus extracts after acidic treatment. Int Arch Allergy Immunol. 2008; 145: 298-304. Ref.: https://goo.gl/azdfme
- Iraola VM, Leonor JR, Morales M, López Matas MA, Gallego MT, Carnés J. Enzymatic Activity of Grass Native Allergenic Extracts Is Eliminated in Depigmented Allergoids. Journal of Allergy and Clinical Immunology. 2013; 131: 110. Ref.: https://goo.gl/Eei5fQ
- Pfaar O, Sager A, Robinson DS. Safety and effect on reported symptoms of depigmented polymerized allergen immunotherapy: a retrospective study of 2927 paediatric patients. Pediatr Allergy Immunol. 2015; 26: 280-286. Ref.: https://goo.gl/EGN6rz
- Johansen P, Senti G, Gómez JMM, Wüthrich B, Bot A, et al. Heat denaturation, a simple method to improve the immunotherapeutic potential of allergens. Eur J Immunol. 2005; 35: 3591-3598. Ref.: https://goo.gl/9ryW7X
- Davis PJ, Williams SC. Protein modification by thermal processing. Allergy. 1998; 53: 102-105. Ref.: https://goo.gl/nxXkWm
- Hildebrandt S, Kratzin HD, Schaller R, Fritsché R, Steinhart H, et al. In Vitro Determination of the Allergenic Potential of Technologically Altered Hen's Egg. J Agric Food Chem. 2008; 56: 1727-1733. Ref.: https://goo.gl/Jhm5Bs
- Kim JS, Nowak-Wegrzyn A, Sicherer SH, Noone S, Moshier EL, et al. Dietary baked milk accelerates the resolution of cow's milk allergy in children. J Allergy Clin Immunol. 2011; 128: 125-131. Ref.: https://goo.gl/LtRSCy
- Beyer K, Morrow E, Li XM, Bardina L, Bannon GA, et al. Effects of cooking methods on peanut allergenicity. J Allergy Clin Immunol. 2001; 107: 1077-1081. Ref.: https://goo.gl/oXEDkY
- Naterman HL. Formalinized pollen tannate in desensitization treatment. J Allergy. 1957; 28: 76-83. Ref.: https://goo.gl/YTt7cF
- Ishizaka K, Okudaira H, King TP. Immunogenic Properties of Modified Antigen E - II. Ability of Urea-Denatured Antigen and alpha-Polypeptide Chain to Prime T Cells Specific for Antigen E. J Immunol. 1975; 114: 110-115. Ref.: https://goo.gl/yFPnWF
- Marsh DG, Norman PS, Roebber M, Lichtenstein LM. Studies on allergoids from naturally occurring allergens III. Preparation of ragweed pollen allergoids by aldehyde modification in two steps. J Allergy Clin Immunol. 1981; 68: 449-459. Ref.: https://goo.gl/V65UeX
- Avrameas S. Coupling of enzymes to proteins with glutaraldehyde. Immunochemistry. 1969; 6: 43-52.
- Avrameas S, Ternynck T. The Cross-linking of Proteins with Glutaraldehyde and its use for the preparation of immunoadsorbents. Immunochemistry. 1969; 6: 53-66. Ref.: https://goo.gl/65CFhq
- Haddad ZH, Marsh DG, Campbell DH. Studies on "allergoids" prepared from naturally occurring allergens II. Evaluation of allergenicity and assay of antigenicity of formalinized mixed grass pollen extracts. J Allergy Clin Immunol. 1972; 49: 197-209. Ref.: https://goo.gl/jn2uPK
- Urry ZL, Richards DF, Black C, Morales M, Carnes J, et al. Depigmented-polymerised allergoids favour regulatory over effector T cells: enhancement by 1-alpha, 25-dihydroxyvitamin D3. BMC Immunol. 2014; 15: 21. Ref.: https://goo.gl/PzdNDC
- Casanovas M, Gómez MJ, Carnés J, Fernández-Caldas E. Skin tests with native, depigmented and glutaraldehyde polymerized allergen extracts. J Invest Allergol Clin Immunol. 2005; 15: 30-36. Ref.: https://goo.gl/uU5k4H
- Norman PS, Lichtenstein LM, Marsh DG. Studies on allergoids from naturally occurring allergens. IV. Efficacy and safety of long-term allergoid treatment of ragweed hay fever. J Allergy Clin Immunol. 1981; 68: 460-470. Ref.: https://goo.gl/fs4jrx
- Kalinski P, Lebre MC, Kramer D, De Jong EC, Van Schijndel JW, et al. Analysis of the CD4+ T cell responses to house dust mite allergoid. Allergy. 2003; 58: 648-656. Ref.: https://goo.gl/yAjJuU
- Salgado J, Casadevall G, Puigneró V, Queralt J. Characterization of Allergoids from Ovalbumin in vitro and in vivo. Immunobiology. 1996; 196: 375-386. Ref.: https://goo.gl/nBiK4B
- Patterson R, Suszko IM, McIntire FC. Polymerized Ragweed Antigen E I. Preparation and Immunologic Studies. J Immunol. 1973; 110: 1402-1412. Ref.: https://goo.gl/us2gBc
- Patterson R, Suszko IM, Pruzansky JJ, Zeiss CR. Polymerized Ragweed Antigen E II. In vivo Elimination Studies and Reactivity with IgE Antibody Systems. J Immunol. 1973; 110: 1413-1418. Ref.: https://goo.gl/Kioi5r
- Patterson R, Suszko IM, Pruzansky JJ, Zeiss CR, Metzger WJ, et al. Polymerization of mixtures of grass allergens. J Allergy Clin Immunol. 1977; 59: 314-319. Ref.: https://goo.gl/LNeHnw
- Gallego MT, Iraola V, Himly M, Robinson DS, Badiola C, et al. Depigmented and polymerised house dust mite allergoid: allergen content, induction of IgG4 and clinical response. Int Arch Allergy Immunol. 2010; 153: 61-69. Ref.: https://goo.gl/N1GRGR
- HayGlass KT, Strejan GH. Antigen- and IgE class-specific suppression mediated by T suppressor cells of mice treated with glutaraldehyde-polymerized ovalbumin. Int Arch Allergy Appl Immunol. 1983; 71: 23-31. Ref.: https://goo.gl/eSGzdA
- Morales M, Gallego M, Iraola V, Taulés M, de Oliveira E, et al. In vitro evidence of efficacy and safety of a polymerized cat dander extract for allergen immunotherapy. BMC Immunol. 2017; 18: 10. Ref.: https://goo.gl/ovxFDV
- Heydenreich B, Bellinghausen I, Lorenz S, Henmar H, Strand D, et al. Reduced in vitro T-cell responses induced by glutaraldehyde-modified allergen extracts are caused mainly by retarded internalization of dendritic cells. Immunology. 2012; 136: 208-217. Ref.: https://goo.gl/aNcFTJ
- Villas-Boas MB, Vieira KP, Trevizan G, Zollner RL, Netto FM. The effect of transglutaminase-induced polymerization in the presence of cysteine on beta-lactoglobulin antigenicity. Int Dairy J. 2010; 20: 386-392. Ref.: https://goo.gl/RdN37z
- Hendrix S, Zeiss CR, Suszko I, Levitz D, Patterson R. Polymerized whole ragweed: An improved method of immunotherapy. J Allergy Clin Immunol. 1978; 62: 289-294. Ref.: https://goo.gl/vvK5Ys
- Mistrello G, Roncarolo D, Gentili M, Zanoni D, Falagiani P. Modified par j I allergen from P. judaica pollen and its rate of absorption in rats. Immunol Lett. 1994; 40: 31-36. Ref.: https://goo.gl/BrLzif
- Akdis CA, Barlan IB, Bahceciler N, Akdis M. Immunological mechanisms of sublingual immunotherapy. Allergy. 2006; 61: 11-14. Ref.: https://goo.gl/qixFuZ
- Manzano AI, Javier Cañada F, Cases B, Sirvent S, Soria I, et al. Structural studies of novel glycoconjugates from polymerized allergens (allergoids) and mannans as allergy vaccines. Glycoconj J. 2016; 33: 93-101. Ref.: https://goo.gl/5wwQQR
- Roger A, Depreux N, Jurgens Y, Heath MD, Garcia G, et al. A novel and well tolerated mite allergoid subcutaneous immunotherapy: evidence of clinical and immunologic efficacy. Immun Inflamm Dis. 2014; 2: 92-98. Ref.: https://goo.gl/kG9DuY
- Gokmen NM, Ersoy R, Gulbahar O, Ardeniz O, Sin A, et al. Desensitization effect of preseasonal seven-injection allergoid immunotherapy with olive pollen on basophil activation: the efficacy of olive pollen-specific preseasonal allergoid immunotherapy on basophils. Int Arch Allergy Immunol. 2012; 159: 75-82. Ref.: https://goo.gl/GvwL8J
- Pfaar O, Sager A, Robinson DS. Safety and effect on reported symptoms of depigmented-polymerised allergen immunotherapy: a retrospective study of 2927 paediatric patients. Pediatr Allergy Immunol. 2015. 26: 280-286. Ref.: https://goo.gl/cUsWgY
- Lozano J, Cruz MJ, Piquer M, Giner MT, Plaza AM. Assessing the Efficacy of Immunotherapy with a Glutaraldehyde-Modified House Dust Mite Extract in Children by Monitoring Changes in Clinical Parameters and Inflammatory Markers in Exhaled Breath. Int Arch Allergy Immunol. 2014; 165: 140-147. Ref.: https://goo.gl/QsZAFC
- Weinberger EE, Himly M, Myschik J, Hauser M, Altmann F, et al. Generation of hypoallergenic neoglycoconjugates for dendritic cell targeted vaccination: a novel tool for specific immunotherapy. J Control Release. 2013; 165: 101-109. Ref.: https://goo.gl/mL2YMf
- Aboumahmoud R, Savello P. Crosslinking of Whey Protein by Transglutaminase. J Dairy Sci. 1990; 73: 256-263. Ref.: https://goo.gl/tv8YTK
- Jaros D, Partschefeld C, Henle T, Rohm H. Transglutaminase in dairy products: chemistry, physics, applications. J Texture Studies. 2006; 37: 113-155. Ref.: https://goo.gl/2pWLyW
- Cozzolino A, Di Pierro P, Mariniello L, Sorrentino A, Masi P, et al. Incorporation of whey proteins into cheese curd by using transglutaminase. Biotechnol Appl Biochem. 2003; 38: 289-295. Ref.: https://goo.gl/8inU3j
- Eissa AS, Puhl C, Kadla JF, Khan SA. Enzymatic Cross-Linking of β-Lactoglobulin: conformational properties using FTIR Spectroscopy. Biomacromolecules. 2006; 7: 1707-1713. Ref.: https://goo.gl/6aovxp
- Greenberg CS, Birckbichler PJ, Rice RH. Transglutaminases: multifunctional cross-linking enzymes that stabilize tissues. FASEB J. 1991; 5: 3071-3077. Ref.: https://goo.gl/wGyHFm
- Pedersen MH, Hansen TK, Sten E, Seguro K, Ohtsuka T, et al. Evaluation of the potential allergenicity of the enzyme microbial transglutaminase using the 2001 FAO/WHO Decision Tree. Mol Nutr Food Res. 2004; 48: 434-440. Ref.: https://goo.gl/2v1ghj
- Olivier CE, Villas-Boas MB, Netto FM, Zollner RL. Allergenicity of Bos d 5 in Children with Cow’s Milk Allergy is Reduced by Transglutaminase Polymerization. Ped Allergy Immunol Pulmonol. 2012; 25: 30-33. Ref.: https://goo.gl/7BUHna
- Giosafatto CVL, Rigby NM, Wellner N, Ridout M, Husband F, et al. Microbial transglutaminase-mediated modification of ovalbumin. Food Hydrocolloids. 2012; 26: 261-267. Ref.: https://goo.gl/rN9PMM
- Villas-Boas MB, Fernandes MA, Zollner RdL, Netto FM. Effect of polymerization with transglutaminase on in vitro digestion and antigenicity of beta-lactoglobulin. Int Dairy J. 2012; 25: 123-131. Ref.: https://goo.gl/G3vyy9
- Damodaran S, Li Y. A two-step enzymatic modification method to reduce immuno-reactivity of milk proteins. Food Chem. 2017; 237: 724-732. Ref.: https://goo.gl/rY5ZmP
- Mistrello G, Brenna O, Roncarolo D, Zanoni D, Gentili M, et al. Monomeric chemically modified allergens: Immunologic and physicochemical characterization. Allergy. 1996; 51: 8-15. Ref.: https://goo.gl/WRFJAf
- Nguyen NT, Raskopf E, Shah-Hosseini K, Zadoyan G, Mösges R. A review of allergoid immunotherapy: is cat allergy a suitable target? Immunotherapy. 2016; 8: 331-349. Ref.: https://goo.gl/CvSvQ2
- Lombardi C, Gargioni S, Melchiorre A, Tiri A, Falagiani P, et al. Safety of sublingual immunotherapy with monomeric allergoid in adults: multicenter post-marketing surveillance study. Allergy. 2001; 56: 989-992. Ref.: https://goo.gl/TL2Egx
- Hüser C, Dieterich P, Singh J, Shah-Hosseini K, Allekotte S, et al. A 12-week DBPC dose-finding study with sublingual monomeric allergoid tablets in house dust mite-allergic patients. Allergy. 2016; 72: 77-84. Ref.: https://goo.gl/F8svNS
- Scalone G, Compalati E, Bruno ME, Mistrello G. Effect of two doses of carbamylated allergoid extract of dust mite on nasal reactivity. Eur Ann Allergy Clin Immunol. 2013; 45: 193-200. Ref.: https://goo.gl/f3dJcX
- Mösges R, Ritter B, Kayoko G, Passali D, Allekotte S. Carbamylated monomeric allergoids as a therapeutic option for sublingual immunotherapy of dust mite- and grass pollen-induced allergic rhinoconjunctivitis: a systematic review of published trials with a meta-analysis of treatment using Lais® tablets. Acta Dermatovenerologica APA. 2010; 19: 3-10. Ref.: https://goo.gl/vfJ3Nr
- Hüser C, Dieterich P, Singh J, Shah-Hosseini K, Allekotte S, et al. A 12‐week DBPC dose‐finding study with sublingual monomeric allergoid tablets in house dust mite‐allergic patients. Allergy. 2017; 72: 77-84. Ref.: https://goo.gl/rFyDj8
- Agostinis F, Tellarini L, Canonica GW, Falagiani P, Passalacqua G. Safety of sublingual immunotherapy with a monomeric allergoid in very young children. Allergy 2005; 60:133. Ref.: https://goo.gl/za4oTu
- D'Anneo RW, Arena A, Gammeri E, Bruno ME, Falagiani P, et al. Parietaria sublingual allergoid immunotherapy with a co-seasonal treatment schedule. Allergologia et Immunopathologia. 2008; 45: 79-84. Ref.: https://goo.gl/wWRmKK
- Shershakova N, Bashkatova E, Babakhin A, Andreev S, Nikonova A, et al. Allergen-Specific Immunotherapy with Monomeric Allergoid in a Mouse Model of Atopic Dermatitis. PLoS ONE. 2015; 10. Ref.: https://goo.gl/4jHorH
- Zivny JH, Moldoveanu Z, Vu HL, Russell MW, Mestecky J, et al. Mechanisms of Immune Tolerance to Food Antigens in Humans. Clin Immunol. 2001; 101: 158-68. Ref.: https://goo.gl/kEtK3Z
- Larché M. Immunotherapy with Allergen Peptides. Allergy, Asthma, and Clinical . 2007; 3: 53-59. Ref.: https://goo.gl/Fm56Pw
- Geysen HM, Rodda SJ, Mason TJ. A priori delineation of a peptide which mimics a discontinuous antigenic determinant. Mol Immunol. 1986; 23:7 09-715. Ref.: https://goo.gl/jQ4C6E
- Sibilano R, Frossi B, Pucillo CE. Mast cell activation: A complex interplay of positive and negative signaling pathways. Eur J Immunol. 2014; 44: 2558-2566. Ref.: https://goo.gl/hwVx3J
- Lambrakis P, Rushworth GF, Adamson J, Leslie SJ. Aspirin hypersensitivity and desensitization protocols: implications for cardiac patients Ther Adv Drug Saf. 2011; 2: 263-270. Ref.: https://goo.gl/LvKewX
- Morris DL. Intradermal testing and sublingual desensitization for nickel. Cutis 1998; 61: 129-132. Ref.: https://goo.gl/DQGo1H
- Majewska-Szczepanik M, Strzepa A, Drozynska I, Motyl S, Banach T, et al. Epicutaneous immunization with hapten-conjugated protein antigen alleviates contact sensitivity mediated by three different types of effector cells. Pharmacological Reports 2012; 64: 919-926. Ref.: https://goo.gl/XRUjXN
- Gadermaier E, James LK, Shamji MH, Blatt K, Fauland K, et al. Epitope specificity determines cross-protection of a SIT-induced IgG(4) antibody. Allergy. 2016; 71: 36-46. Ref.: https://goo.gl/ZCuqWf
- Luzar J, Štrukelj B, Lunder M. Phage display peptide libraries in molecular allergology: from epitope mapping to mimotope-based immunotherapy. Allergy. 2016; 71: 1526-1532. Ref.: https://goo.gl/hWW7N1
- Olivier CE. Food Allergy. J Aller Ther. 2013; S3: 1-7. Ref.: https://goo.gl/ztUVpk
- Untersmayr E, Scholl I, Swoboda I, Beil WJ, Forster-Waldl E, et al. Antacid medication inhibits digestion of dietary proteins and causes food allergy: a fish allergy model in BALB/c mice. J Allergy Clin Immunol. 2003; 112: 616-623. Ref.: https://goo.gl/2gZ5Tt
- Pecquet S, Bovetto L, Maynard F, Fritsché R. Peptides obtained by tryptic hydrolysis of bovine Beta-lactoglobulin induce specific oral tolerance in mice J Allergy Clin Immunol. 2000; 105: 514-521. Ref.: https://goo.gl/dVhfQg
- Astwood JD, Leach JN, Fuchs RL. Stability of food allergens to digestion in vitro. Nat Biotechnol. 1996; 14: 1269-1273. Ref.: https://goo.gl/GQP8Es
- Lucas JS, Cochrane SA, Warner JO, Hourihane JO. The effect of digestion and pH on the allergenicity of kiwifruit proteins. Pediatr Allergy Immunol. 2008; 19: 392-398. Ref.: https://goo.gl/dFfop6
- Untersmayr E, Poulsen LK, Platzer MH, Pedersen MH, Boltz-Nitulescu, et al. The effects of gastric digestion on codfish allergenicity. J Allergy Clin Immunol. 2005; 115: 377-382. Ref.: https://goo.gl/NG5TLA
- Olivier CE, Lima RPdS, Argentão DGP, Silva MDd, Santos RAPGd, et al. The immunodominant allergen of genetically improved farmed tilapia (Oreochromis niloticus)is a 100-kDa protein susceptible to pepsin digestion in four fish-allergic Brazilian patients. Braz J Aller Immunol. 2015; 3: 261-265.
- Tavano OL. Protein hydrolysis using proteases: An important tool for food biotechnology. J Molecular Catalysis B: Enzymatic. 2013; 90: 1-11. Ref.: https://goo.gl/EvVYov
- Fritsché R. Induction of oral tolerance to cow's milk proteins in rats fed with a whey protein hydrolysate. Nutrition Research. 1998; 18: 1335-1341. Ref.: https://goo.gl/EBUExX
- Hacini-Rachinel F, Vissers YM, Doucet-Ladevéze R, Blanchard C, Demont A, et al. Low-Allergenic Hydrolyzed Egg Induces Oral Tolerance in Mice. Int Arch Aller Immunol. 2014; 164: 64-73. Ref.: https://goo.gl/9wLxku
- Esch BCAMv, Schouten B, Kivit Sd, Hofman GA, Knippels, et al. Oral tolerance induction by partially hydrolyzed whey protein in mice is associated with enhanced numbers of Foxp3+ regulatory T-cells in the mesenteric lymph nodes. Pediatr Allergy Immunol. 2011; 22: 820-826. Ref.: https://goo.gl/srhqbU
- Fritsché R, Pahud JJ, Pecquet S, Pfeifer A. Induction of systemic immunologic tolerance to beta-lactoglobulin by oral administration of a whey protein hydrolysate. J Aller Clinical Immunol. 1997; 100: 266-273. Ref.: https://goo.gl/c2fyT5
- Yang M, Yang C, Nau Fo, Pasco M, Juneja LR, et al. Immunomodulatory Effects of Egg White Enzymatic Hydrolysates Containing Immunodominant Epitopes in a BALB/c Mouse Model of Egg Allergy. J Agricul Food Chem. 2009; 57: 2241-2248. Ref.: https://goo.gl/mRDpFG
- Vaarala O, Saukkonen T, Savilahti E, Klemola T, Akerblom HK. Development of immune response to cow's milk proteins in infants receiving cow's milk or hydrolyzed formula. J Aller Clinical Immunol. 1995; 96: 917-923. Ref.: https://goo.gl/z37dcz
- Lanzavecchia A, Frühwirth A, Perez L, Corti D. Antibody-guided vaccine design: identification of protective epitopes. Curr Opin Immunol. 2016; 41: 62-67. Ref.: https://goo.gl/HFZHaL
- de Lalla C, Sturniolo T, Abbruzzese L, Hammer J, Sidoli A, et al. Identification of Novel T Cell Epitopes in Lol p5a by Computational Prediction. J Immunol. 1999; 163: 1725-1729. Ref.: https://goo.gl/1yX5Wf
- Nielsen M, Lundegaard C, Worning P, Hvid CS, Lamberth K, et al. Improved prediction of MHC class I and class II epitopes using a novel Gibbs sampling approach. Bioinformatics. 2004; 20: 1388-1397. Ref.: https://goo.gl/Nb2Wkr
- Dhiraj H, Paul RY, David PF. Current status of short synthetic peptides as vaccines. Med Chem. 2006; 2: 627-646. Ref.: https://goo.gl/jmXwPN
- Merrifield RB. Solid Phase Peptide Synthesis. I. The Synthesis of a Tetrapeptide. J American Chem Society. 1963; 85: 2149-2154. Ref.: https://goo.gl/AiZywi
- Geysen HM, Meloen RH, Barteling SJ. Use of peptide synthesis to probe viral antigens for epitopes to a resolution of a single amino acid. Proc Natl Acad Sci U S A. 1984; 81: 3998-4002. Ref.: https://goo.gl/tVhwS6
- Sumar N. Multiple pin peptide scanning ("Pepscan"). In: Westwood OMR, Hay FC, editors. Epitope mapping -A Pratical approach. 2001; 17-42.
- Roggero MA, Servis C, Corradin G. A simple and rapid procedure for the purification of synthetic polypeptides by a combination of affinity chromatography and methionine chemistry. Febs Letters. 1997; 408: 285-288. Ref.: https://goo.gl/QEJADG
- Fellrath J-M, Kettner A, Dufour N, Frigerio C, Schneeberger D, et al. Allergen-specific T-cell tolerance induction with allergen-derived long synthetic peptides: Results of a phase I trial. J Aller Clinical Immunol. 2003; 111: 854-861. Ref.: https://goo.gl/bZMnZ3
- Maguire P, Nicodemus C, Robinson D, Aaronson D, Umetsu DT. The Safety and Efficacy of ALLERVAX CAT in Cat Allergic Patients. Clin Immunol. 1999; 93: 222-231. Ref.: https://goo.gl/qL1TrV
- Norman PS, Jr JLO, Long AA, Creticos PS, Gefter MA, et al. Treatment of cat allergy with T-cell reactive peptides. Am J Respir Crit Care Med. 1996; 154: 1623-1628. Ref.: https://goo.gl/qZhkSs
- Müller U, Akdis CA, Fricker M, Akdis M, Blesken T, et al. Successful immunotherapy with T-cell epitope peptides of bee venom phospholipase A2 induces specific T-cell anergy in patients allergic to bee venom. J Allergy Clin Immunol. 1998; 101: 747-754. Ref.: https://goo.gl/7wStu9
- Marcotte GV, Braun CM, Norman PS, Nicodemus CF, Kagey-Sobotka A, et al. Effects of peptide therapy on ex vivo T-cell responses. J Allergy Clinical Immunol. 1998; 101: 506-513. Ref.: https://goo.gl/mkckrx
- Oldfield WLG, Larché M, Kay AB. Effect of T-cell peptides derived from Fel d 1 on allergic reactions and cytokine production in patients sensitive to cats: a randomised controlled trial. Lancet . 2002; 360: 47-53. Ref.: https://goo.gl/t7S4qT
- Pellaton C, Perrin Y, Boudousquié C, Barbier N, Wassenberg J, et al. Novel birch pollen specific immunotherapy formulation based on contiguous overlapping peptides. Clinical and Translational Allergy. 2013; 3: 17. Ref.: https://goo.gl/9aaBXc
- Spertini F, Perrin Y, Audran R, Pellaton C, Boudousquié C, et al. Safety and immunogenicity of immunotherapy with Bet v 1 derived contiguous overlapping peptides. J Aller Clinical Immunol. 2014; 134: 239-240. Ref.: https://goo.gl/qPPi38
- Spertini F, DellaCorte G, Kettner A, de Blay F, Jacobsen L, et al. Efficacy of 2 months of allergen-specific immunotherapy with Bet v 1-derived contiguous overlapping peptides in patients with allergic rhinoconjunctivitis: Results of a phase IIb study. J Aller Clinical Immunol. 2016; 138: 162-168. Ref.: https://goo.gl/WidJ2w
- Stephen SL, Sivanandam VG, Kochanek S. Homologous and heterologous recombination between adenovirus vector DNA and chromosomal DNA. J Gene Medicine. 2008; 10: 1176-1189. Ref.: https://goo.gl/gaCZho
- Chen K-W, Blatt K, Thomas WR, Swoboda I, Valent P, et al. Hypoallergenic Der p 1/Der p 2 combination vaccines for immunotherapy of house dust mite allergy. J Allergy Clin Immunol. 2012; 130: 435-443. Ref.: https://goo.gl/5DfTFL
- Bouchaud G, Braza F, Chesnà J, Lair D, Chen K-W, et al. Prevention of allergic asthma through Der p 2 peptide vaccination. J Allergy Clin Immunol. 2015; 136: 197-200. Ref.: https://goo.gl/LcWHqr
- Ferreira F, Hirthenlehner K, Briza P, Breiteneder H, Scheiner O, et al. Isoforms of atopic allergens with reduced allergenicity but conserved T cell antigenicity: possible use for specific immunotherapy. Int Arch Allergy Immunol. 1997; 113: 125-127. Ref.: https://goo.gl/mFwSYP
- Zaborsky N, Brunner M, Wallner M, Himly M, Karl T, et al. Antigen Aggregation Decides the Fate of the Allergic Immune Response. J Immunol. 2010; 184: 725-735. Ref.: https://goo.gl/1rHNJ9
- Chen KW, Focke-Tejkl M, Blatt K, Kneidinger M, Gieras A, et al. Carrier-bound nonallergenic Der p 2 peptides induce IgG antibodies blocking allergen-induced basophil activation in allergic patients. Allergy. 2012; 67: 609-621. Ref.: https://goo.gl/zWFxLB
- Wiedermann U, Jahn-Schmid B, Lindblad M, Rask C, Holmgren J, et al. Suppressive versus stimulatory effects of allergen/cholera toxoid (CTB) conjugates depending on the nature of the allergen in a murine model of type I allergy. Int Immunol. 1999; 11: 1131-1138. Ref.: https://goo.gl/aVLbpH
- Cornelius C, Schöneweis K, Georgi F, Weber M, Niederberger V, et al. Immunotherapy With the PreS-based Grass Pollen Allergy Vaccine BM32 Induces Antibody Responses Protecting Against Hepatitis B Infection. EBioMedicine. 2016; 11: 58-67. Ref.: https://goo.gl/rLFeci
- Pichler U, Hauser M, Hofer H, Himly M, Hoflehner E, et al. Allergen hybrids - next generation vaccines for Fagales pollen immunotherapy. Clin Exp Allergy. 2014; 44: 438-449. Ref.: https://goo.gl/9mMJCs
- Wheeler AW, Woroniecki SR. Immunological adjuvants in allergy vaccines: Past, present future. Int Allergology. 2001; 50: 295-301. Ref.: https://goo.gl/jBGBMZ
- Apostolopoulos V, Thalhammer T, Tzakos AG, Stojanovska L. Targeting Antigens to Dendritic Cell Receptors for Vaccine Development. J Drug Deliv. 2013; 2013: 869718. Ref.: https://goo.gl/a4ox2r
- Kahlert H, Grage-Griebenow E, Stuwe HT, Cromwell O, Fiebig H. T Cell Reactivity with Allergoids: Influence of the type of APC. J Immunol. 2000; 165: 1807-1815. Ref.: https://goo.gl/Ymnx54
- Vlad G, Cortesini R, Suciu-Foca N. License to Heal: Bidirectional Interaction of Antigen-Specific Regulatory T Cells and Tolerogenic APC. J Immunol. 2005; 174: 5907-5914. Ref.: https://goo.gl/YHdjsz
- Salazar F, Sewell HF, Shakib F, Ghaemmaghami AM. The role of lectins in allergic sensitization and allergic disease. J Allergy Clin Immunol. 2013; 132: 27-36. Ref.: https://goo.gl/fU5z6h
- Figdor CG, van Kooyk Y, Adema GJ. C-type lectin receptors on dendritic cells and langerhans cells. Nat Rev Immunol. 2002; 2: 77-84. Ref.: https://goo.gl/sjqHYs
- Leroux-Roels G, Marchant A, Levy J, Van Damme P, Schwarz TF, et al. Impact of adjuvants on CD4+ T cell and B cell responses to a protein antigen vaccine: Results from a phase II, randomized, multicenter trial. Clin Immunol. 2016; 169: 16-27. Ref.: https://goo.gl/G9jYkn
- Burny W, Callegaro A, Bechtold V, Clement F, Delhaye S, et al. Different Adjuvants Induce Common Innate Pathways That Are Associated with Enhanced Adaptive Responses against a Model Antigen in Humans. Front Immunol. 2017; 8: 1-17. Ref.: https://goo.gl/3Q9Ktq
- Lipton MM, Freund J. The formation of complement fixing and neutralizing antibodies after the injection of inactivated rabies virus with adjuvants. J Immunol. 1950; 64: 297-303. Ref.: https://goo.gl/xVRGCs
- Kearney SC, Dziekiewicz M, Feleszko W. Immunoregulatory and immunostimulatory responses of bacterial lysates in respiratory infections and asthma. Ann Allergy Asthma Immunol. 2015; 114: 364-369. Ref.: https://goo.gl/z4dMdB
- Ahrens B, Quarcoo D, Buhner S, Matricardi PM, Hamelmann E. Oral Administration of Bacterial Lysates Attenuates Experimental Food Allergy. Int Arch Allergy Immunol. 2011; 156: 196-204. Ref.: https://goo.gl/5qMnVj
- Navarro S, Cossalter G, Chiavaroli C, Kanda A, Fleury S, et al. The oral administration of bacterial extracts prevents asthma via the recruitment of regulatory T cells to the airways. Mucosal Immunol. 2011; 4: 53-65. Ref.: https://goo.gl/BBA9j2
- Xu LZ, Yang LT, Qiu SQ, Yang G, Luo XQ, et al. Combination of specific allergen and probiotics induces specific regulatory B cells and enhances specific immunotherapy effect on allergic rhinitis. Oncotarget. 2016; 7: 54360-54369. Ref.: https://goo.gl/eW1XVH
- Glenny AT, Barr M. The precipitation of diphtheria toxoid by potash alum. J Pathol Bacteriol. 1931; 34: 131-138. Ref.: https://goo.gl/YrCVM7
- Sledge RF. Treatment of hay fever with alum-precipitated pollen extract. J Allergy. 1938; 9: 424.
- Mannhalter JW, Neychev HO, Zlabinger GJ, Ahmad R, Eibl MM. Modulation of the human immune response by the non-toxic and non-pyrogenic adjuvant aluminium hydroxide: effect on antigen uptake and antigen presentation. Clin Exp Immunol. 1985; 61: 143-151. Ref.: https://goo.gl/GmTjx9
- Jones SK, Lovell CR, Peachey RD. Delayed onset of inflammatory nodules following hay fever desensitization injections. Clin Exp Dermatol. 1988; 13: 376-378. Ref.: https://goo.gl/iX6zbF
- Castelain PY, Castelain M, Vervloet D, Garbe L, Mallet B. Sensitization to aluminium by aluminium-precipitated dust and pollen extracts. Contact Dermatitis. 1988; 19: 58-60. Ref.: https://goo.gl/aKTg4H
- Veien NK, Hattel T, Justesen O, Norholm A. Aluminium allergy. Contact Dermatitis. 1986; 15: 295-297. Ref.: https://goo.gl/N5B2hf
- Bondy SC, Campbell A. Oxidative and Inflammatory Properties of Aluminum: Possible Relevance in Alzheimer's Disease. In: Exley C, editor. Aluminium and Alzheimer's Disease. Amsterdam. 2001; 311-321.
- Pfaar O, Hohlfeld JM, Al-Kadah B, Hauswald B, Homey B, et al. Dose‐response relationship of a new Timothy grass pollen allergoid in comparison to a 6‐grass pollen allergoid. Clin Exp Allergy. 2017. Ref.: https://goo.gl/GgJQDz
- Kepil Özdemir S, Sin BA, Güloğlu D, İkincioğulları A, Gençtürk Z, et al. Short-term preseasonal Immunotherapy: is early clinical efficacy related to the Basophil Response? Int Arch Allergy Immunol. 2014; 164: 237-245. Ref.: https://goo.gl/kCQTeo
- Heydenreich B, Bellinghausen I, Lund L, Henmar H, Lund G, et al. Adjuvant effects of aluminium hydroxide-adsorbed allergens and allergoids – differences in vivo and in vitro. Clin Exp Immunol. 2014; 176: 310-319. Ref.: https://goo.gl/o9UFqb
- Wheeler AW, Whittall N, Spackman V, Moran DM. Adjuvant Properties of Hydrophobic Derivatives Prepared from L-Tyrosine. Int Arch Allergy Immunol. 1984; 75: 294-299. Ref.: https://goo.gl/MJWKBg
- Wheeler AW, Moran DM, Robins BE, Driscoll A. L-Tyrosine as an Immunological Adjuvant. Int Arch Allergy Immunol. 1982; 69: 113-119. Ref.: https://goo.gl/h92rNb
- Bell AJ, Heath MD, Hewings SJ, Skinner MA. The adsorption of allergoids and 3-O-desacyl-4'-monophosphoryl lipid A (MPLA®) to microcrystalline tyrosine (MCT) in formulations for use in allergy immunotherapy. J Inorg Biochem. 2015; 152: 147-153. Ref.: https://goo.gl/kxR6RL
- Baldrick P, Richardson D, Wheeler AW. Review of L-tyrosine confirming its safe human use as an adjuvant. J Appl Toxicol. 2002; 22: 333-344. Ref: https://goo.gl/KquyrL
- Roger A, Depreux N, Jurgens Y, Serra AT, Heath MD, et al. A novel microcrystalline tyrosine-adsorbed, mite-allergoid subcutaneous immunotherapy: 1-year follow-up report. Immunotherapy. 2016; 8: 1169-1174. Ref.: https://goo.gl/z1XEj3
- Sheng KC, Pouniotis DS, Wright MD, Kit Tang C, Lazoura E, et al. Mannan derivatives induce phenotypic and functional maturation of mouse dendritic cells. Immunology. 2006; 118: 372-383. Ref.: https://goo.gl/BgxzVZ
- Sirvent S, Soria I, Cirauqui C, Cases B, Manzano AI, et al. Novel vaccines targeting dendritic cells by coupling allergoids to nonoxidized mannan enhance allergen uptake and induce functional regulatory Tcells through programmed death ligand 1. J Allergy Clin Immunol. 2016; 138: 558-567. Ref.: https://goo.gl/ZsG57N
- Beutler B, Rietschel ET. Innate immune sensing and its roots: the story of endotoxin. Nat Rev Immunol. 2003; 3: 169-176. Ref.: https://goo.gl/7TFKsA
- Ribi E, Parker R, Strain SM, Mizuno Y, Nowotny A, et al. Peptides as requirement for immunotherapy of the guinea-pig line-10 tumor with endotoxins. Cancer Immunol Immunother. 1979; 7: 43-58. Ref.: https://goo.gl/cgrkYC
- Casella CR, Mitchell TC. Putting endotoxin to work for us: monophosphoryl lipid A as a safe and effective vaccine adjuvant. Cell mol life sci. 2008; 65: 3231-3240. Ref.: https://goo.gl/hpEUEL
- Baldrick P, Richardson D, Woroniecki SR, Lees B. Pollinex Quattro Ragweed: safety evaluation of a new allergy vaccine adjuvanted with monophosphoryl lipid A (MPL) for the treatment of ragweed pollen allergy. J Appl Toxicol. 2007; 27: 399-409. Ref.: https://goo.gl/tkkpqC
- Baldrick P, Richardson D, Wheeler AW. Safety evaluation of a glutaraldehyde modified tyrosine adsorbed house dust mite extract containing monophosphoryl lipid A (MPL®) adjuvant: a new allergy vaccine for dust mite allergy. Vaccine. 2001; 20: 737-743. Ref.: https://goo.gl/FRiUeu
- Drachenberg KJ, Wheeler AW, Stuebner P, Horak F. A well-tolerated grass pollen-specific allergy vaccine containing a novel adjuvant, monophosphoryl lipid A, reduces allergic symptoms after only four preseasonal injections. Allergy. 2001; 56: 498-505. Ref.: https://goo.gl/Kf9F3Z
- Drachenberg KJ, Heinzkill M, Urban E, Woroniecki SR. Efficacy and tolerability of short-term specific immunotherapy with pollen allergoids adjuvanted by monophosphoryl lipid A (MPL®) for children and adolescents. Allergologia et Immunopathologia. 2003; 31: 270-277. Ref.: https://goo.gl/ZaA64x
- Mothes N, Heinzkill M, Drachenberg KJ, Sperr WR, Krauth MT, et al. Allergen-specific immunotherapy with a monophosphoryl lipid A adjuvanted vaccine: reduced seasonally boosted immunoglobulin E production and inhibition of basophil histamine release by therapy induced blocking antibodies. Clin Exp Allergy. 2003; 33: 1198-1208. Ref.: https://goo.gl/LuyGYX
- Worm M, Ernst D, Kraller M, Babina M. The Impact on Allergy-Related Cells of a Birch Pollen Allergoid, with and without Monophosphoryl Lipid A, in Comparison with the Native Equivalent. Int Arch Allergy Immunol. 2017; 172: 20-26. Ref.: https://goo.gl/BrfCYj
- Ellis AK, Tsitoura DC, Quint D, Powley W, Lee LA. Safety and pharmacodynamics of intranasal GSK2245035, a TLR7 agonist for allergic rhinitis: A randomized trial. Clin Exp Allergy. 2017; 47: 1193-1203. Ref.: https://goo.gl/E7V3Zk
- Tighe H, Takabayashi K, Schwartz D, Marsden R, Beck L, et al. Conjugation of protein to immunostimulatory DNA results in a rapid, long-lasting and potent induction of cell-mediated and humoral immunity. Eur J Immunol. 2000; 30: 1939-1947. Ref.: https://goo.gl/MpDKrN
- Tighe H, Takabayashi K, Schwartz D, Van Nest G, Tuck S, et al. Conjugation of immunostimulatory DNA to the short ragweed allergen Amb a 1 enhances its immunogenicity and reduces its allergenicity. J Allergy Clin Immunol. 2000; 106: 124-134. Ref.: https://goo.gl/9VSdBW
- Marshall JD, Abtahi S, Eiden JJ, Tuck S, Milley R, et al. Immunostimulatory sequence DNA linked to the Amb a 1 allergen promotes TH1 cytokine expression while downregulating TH2 cytokine expression in PBMCs from human patients with ragweed allergy. J Allergy Clin Immunol. 2001; 108:191-197. Ref.: https://goo.gl/q1BnQ9
- Creticos PS, Schroeder JT, Hamilton RG, Balcer-Whaley SL, Khattignavong AP, et al. Immunotherapy with a ragweed-toll-like receptor 9 agonist vaccine for allergic rhinitis. N Engl J Med. 2006; 355: 1445-1455. Ref.: https://goo.gl/qFhJCt
- Senti G, Johansen P, Haug S, Bull C, Gottschaller C, et al. Use of A-type CpG oligodeoxynucleotides as an adjuvant in allergen-specific immunotherapy in humans: a phase I/IIa clinical trial. Clin Exp Allergy. 2009; 39: 562-570. Ref.: https://goo.gl/LH9iye
- Levy JH. Anti-inflammatory strategies and hemostatic agents: old Drugs, new Ideas. Hematol Oncol Clin North Am. 2007; 21: 89-101. Ref.: https://goo.gl/4ZiRhk
- Alkjaersig N, Fletcher AP, Sherry S. xi-Aminocaproic acid: an inhibitor of plasminogen activation. J Biol Chem. 1959; 234: 832-837. Ref.: https://goo.gl/KCE9bW
- Camões S, Mendonça I, Marinho JB. Treatment for desensitizing allergic disorders associated with oral epsilon aminocaproic acid. Patologia Geral Brazil. 1971; 56: 87-93.
- Petrarca C, Clemente E, Amato V, Gatta A, Cortese S, et al. Vitamin D3 improves the effects of low dose Der p 2 allergoid treatment in Der p 2 sensitized BALB/c mice. Clin Mol Allergy. 2016; 14: 7. Ref.: https://goo.gl/U8rRhM
- Kirchner B, Pfaffl MW, Dumpler J, von Mutius E, Ege MJ. microRNA in native and processed cow's milk and its implication for the farm milk effect on asthma. J Allergy Clin Immunol. 2016; 137: 1893-1895. Ref.: https://goo.gl/vpcjAa
- Kosaka N, Izumi H, Sekine K, Ochiya T. microRNA as a new immune-regulatory agent in breast milk. Silence. 2010; 1: 7. Ref.: https://goo.gl/cQ3Agt
- Kirchner B, Pfaffl MW, Dumpler J, von Mutius E, Ege MJ. MicroRNA in native and processed cow's milk and its implication for the farm milk effect on asthma. J Allergy Clin Immunol. 2016; 137: 1893-1895. Ref.: https://goo.gl/ghHiR9
- Baier SR, Nguyen C, Xie F, Wood JR, Zempleni J. MicroRNAs Are Absorbed in Biologically Meaningful Amounts from Nutritionally Relevant Doses of Cow Milk and Affect Gene Expression in Peripheral Blood Mononuclear Cells, HEK-293 Kidney Cell Cultures, and Mouse Livers. J Nutr. 2014; 144: 1495-1500. Ref.: https://goo.gl/jw262K
- Melnik BC, John SM, Schmitz G. Milk: An epigenetic inducer of FoxP3 expression. J Allergy Clin Immunol. 2017; 138: 937-938. Ref.: https://goo.gl/oLGebX
- Caubet JC, Bencharitiwong R, Ross A, Sampson HA, Berin MC, et al. Humoral and cellular responses to casein in patients with food protein-induced enterocolitis to cow's milk. J Allergy Clin Immunol. 2017; 139: 572-583. Ref.: https://goo.gl/Q2HWcD
- Krook H, Hagberg A, Song Z, Landegren U, Wennberg L, et al. A distinct Th1 Immune Response Precedes the Described Th2 Response in Islet Xenograft Rejection. Diabetes. 2002; 51: 79-86. Ref.: https://goo.gl/mS1eP1